Biochar application has been shown to benefit soil fertility and to reduce greenhouse gas emissions, thus promoting sustainable and climate-smart agriculture. An important aspect of that sustainability is related to nitrogen availability, which contributes to crop yields but may also cause negative environmental consequences, such as soil nitrous oxide emissions, or leaching of nitrate, and subsequent eutrophication of water systems in the catchment area. In this study, we present results outlining the retention and release of nitrogen by biochar and its effects on nitrogen availability to plants.
KENNETH PELTOKANGAS1,2,3, NELLI PITKÄNEN2, SANNA KANERVA2, MARI PIHLATIE2,3,4
1Finnish Meteorological Institute
2Department of Agricultural Sciences, University of Helsinki, Finland
3Institute for Atmospheric and Earth System Research (INAR), University of Helsinki, Finland
4Viikki Plant Science Centre (ViPS), Department of Agricultural Sciences, University of Helsinki, Finland
Biochar (BC) is currently the most promising agricultural practice for carbon (C) sequestration (Bai et al. 2019). In addition to being composed mainly of recalcitrant C, which can persist in soils for decades (Heikkinen et al. 2021), its application has been shown to benefit soil fertility (Dai et al. 2020) by increasing water and nutrient retention and limiting soil nitrogen (N) losses by reducing nitrous oxide emissions and nitrate (NO3–) leaching (Borchard et al. 2019). However, many studies have provided simple phenomenological assessment of the effects of BC application, leading to limited mechanistic understanding of BC related N retention despite its great environmental significance.
Our aim was to test whether two commercial BCs would exhibit NO3– retention and whether their use would influence N availability to plants. We hypothesized that the retention of NO3– is determined by the physical properties of BC, mainly hydrophobicity and internal porosity. To test our hypotheses, we conducted two parallel experiments in summer 2021. Retention potential and release of mineral-N (NH4+ + NO3–) were studied using consecutive extractions for NO3–-saturated BCs, and a subsequent growth experiment was conducted to examine whether NO3–-saturated BC would cause N deficiency in barley when used as the sole N-source.
Biochars. The study included two commercial BCs: A walnut shell BC (NshBC) and a spruce chip BC (SprBC) produced using flash pyrolysis (800–900 °C) and continuous slow pyrolysis (600 °C), respectively. Both BCs had similar specific surface area (SSA), bulk density (BD), as well as C and N contents, while major differences were in dry weight (DW), particle size distribution, water holding capacity (WHC), pH, and electrical conductivity (Table 1).

Nitrogen retention. The experiment was conducted using NO3–-saturated BC. At first, 5 g (dw) of both BCs (n = 4) were incubated (+4 °C, 93 days) with 10 ml KNO3-solution containing 20 µg N g–1 BC. The incubation was done to ensure that the hydrophobic BC absorbed the KNO3-solution. The BCs were then subjected to three consecutive extractions using 50 ml 2 M KCl-solution, and the extracts were analysed for NH4+-N and NO3–-N using GalleryTM Plus Beermaster Discrete Analyzer (Thermo Scientific, USA).
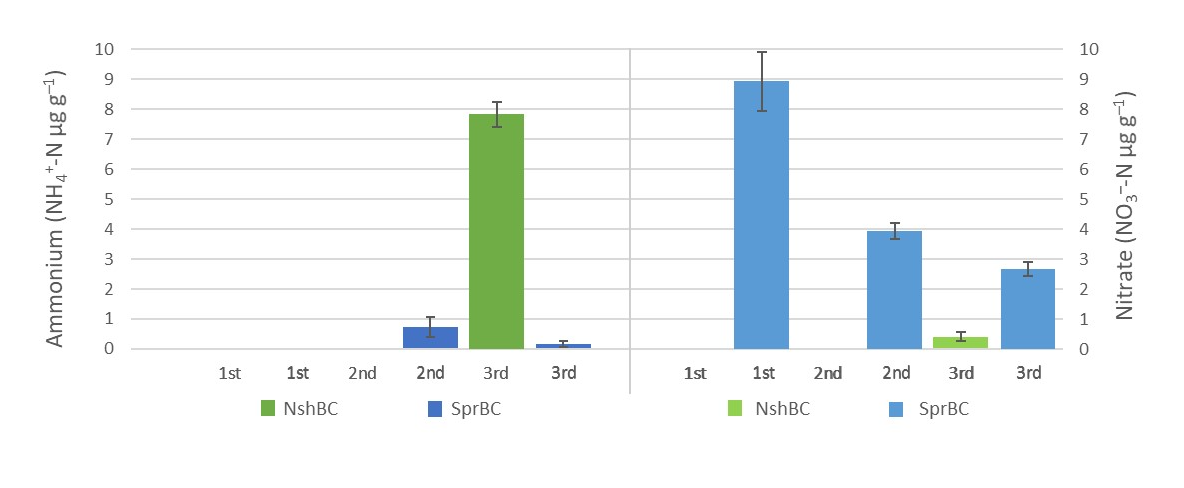
Both BCs initially contained mineral-N equal to approximately 10 % of the added NO3–-N. It is therefore interesting that the recovery rate of NO3–-N from NshBC was less than 2 %, while the recovery rate from SprBC was approximately 80 % (Fig. 1). In both cases, additional extractions would have likely increased the recovery of NO3– as NshBC only started to release mineral-N and the release from SprBC had not yet plateaued. Furthermore, while the amount of NH4+-N in the first extracts was below detection limit (Fig. 1), subsequent extractions released NH4+ from both BCs. In case of NshBC, the amount of NH4+-N released was substantial compared to the total amount of released NO3–-N.
Plant growth. The experiment was conducted in a greenhouse using the two BCs, which were pre-incubated (+4 °C, 14 days) with 5 ml KNO3-solution containing either 20 or 35 µg N g–1 BC. The BCs were mixed with quartz sand in two rates (2 % and 10 % of the volume, n = 4) and the mixture was administered to plastic pots (640 cm3). The fertilization treatments corresponded to 80 and 140 kg N ha–1 (80N and 140N) while the BC application rates were 0 (no BC, only N added as KNO3), 20 (2 % BC), and 100 (10 % BC) Mg ha–1, respectively.
Randomly arranged pots were sown with five pre-germinated barley seeds 3 cm apart and 1 cm deep. Watering was conducted using the watering plates. The plants were fertilized three times with Vita Solatrel (Yara, Finland) during the 36 days experiment. Plant height was measured from the base of the shoots (Fig. 2) and chlorophyll content determined using SPAD-502Plus (Konica Minolta, Japan) three times a week from three individual plants per pot. At the end of the experiment, all shoots were cut, and roots washed. Both roots and shoots were then dried at 40 °C and analysed for their C and N content using 828CN analyser, (LECO, Germany).
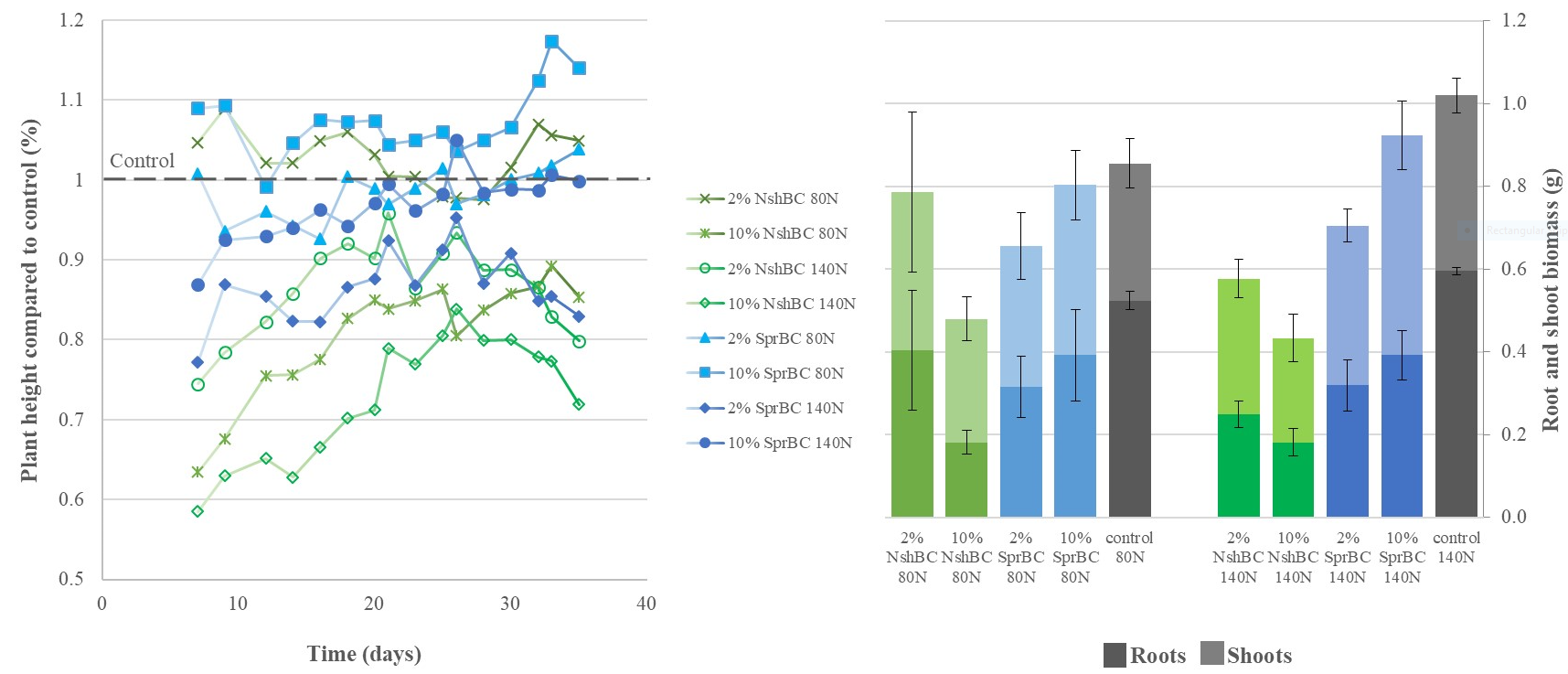
The fertilization rate did not have a significant effect (p > 0.05) on plant height, but combined BC application rate with BC type did (p < 0.05). Plant height was highest with 10 % SprBC + 80N, whereas 10 % NshBC + 140N resulted in the lowest height (Fig. 2). NshBC had the lowest root and shoot biomass, whereas the largest root biomass was observed with the control treatment with 140N fertilization rate. However, the largest shoot biomass was observed with 10 % SprBC 140N (Fig. 2). SprBC treatments had also significantly more chlorophyll than the other treatments until day 25, but at the end of the experiment most BC treatments showed higher N and chlorophyll contents than the control (data not shown).
Future directions. NshBC exhibited significant NO3– retention potential compared to SprBC (Fig. 1). The differences in retention are likely due to the differences in hydrophobicity and wetting properties. In general, hydrophobicity increases with increasing production temperature, and this is reflected by the higher DW and lower WHC of NshBC compared to SprBC (Table 1). Regardless, the experiment demonstrated that future studies involving BC should use repeated extractions to determine mineral-N as the standard method used for extracting soil mineral-N clearly underestimates N retention by BC (Kammann et al. 2015, Haider et al. 2016, Hagemann et al. 2017).
None of the treatments exhibited signs of chlorosis, which would have indicated N deficiency. Furthermore, fertilization rate did not affect growth indicating that N availability was not a growth-limiting factor during the experiment. This is in line with previous studies, as BC has rarely been shown to increase yields, but in this case, N retention did not inhibit growth either. All treatments exhibited yellowing of the shoot tips indicating water stress, likely due to inadequate watering during the hot summer in 2021. The BC treatments did not seem to alleviate water stress despite their high WHC (Table 1). This was likely due to quartz sand, which was chosen as an inert growth medium that contains no N. However, we found that because of its high packing density, it retains water efficiently, but has limited aeration. Mixing it with fine textured BC may have made the situation worse by causing even tighter packing, which could explain the stunted growth by NshBC (Fig. 2). Therefore, future studies should carefully consider the physical properties of the growth medium. Furthermore, despite their distinct particle distributions, both BCs had equal SSA (Table 1), which was also unaffected by grinding. This indicates that SSA was not determined by the external surfaces of the BC particles but their internal pores instead. Therefore, administering BC as large particles may be preferable (Schmidt et al. 2021), as fine textured BC can increase soil BD (Devereux et al. 2012), or when applied to coarse textured soil, they may be susceptible to leaching (Tammeorg et al. 2014), which can limit its value as a soil amendment.
Acknowledgements. The study was conducted in collaboration with Carbo Culture Oy and Carbofex Oy. We thank them for providing us with the studied materials. The project was funded from the joint Carbo Soil -project by University of Helsinki and Carbo Culture Oy (project number 4708998). We also acknowledge Academy of Finland via Flagship program for Atmospheric and Climate Competence Center (ACCC, Grant no 337552)
References
Bai, X., and Coauthors, 2019: Responses of soil carbon sequestration to climate‐smart agriculture practices: A meta‐analysis. Global change biology, 25(8), 2591–2606, https://doi.org/10.1111/gcb.14658.
Borchard, N., and Coauthors, 2019: Biochar, soil and land-use interactions that reduce nitrate leaching and N2O emissions: a meta-analysis. Science of the Total Environment, 651, 2354–2364, https://doi.org/10.1016/j.scitotenv.2018.10.060.
Dai, Y., H. Zheng, Z. Jiang, and B. Xing, 2020: Combined effects of biochar properties and soil conditions on plant growth: a meta-analysis. Science of the total environment, 713, 136635, https://doi.org/10.1016/j.scitotenv.2020.136635.
Devereux, R. C., C. J. Sturrock, and S. J. Mooney, 2012: The effects of biochar on soil physical properties and winter wheat growth. Earth and Environmental Science Transactions of the Royal Society of Edinburgh, 103(1), 13–18, https://doi.org/10.1017/S1755691012000011.
Hagemann, N., C. I. Kammann, H. P. Schmidt, A. Kappler, and S. Behrens, 2017: Nitrate capture and slow release in biochar amended compost and soil. PloS one, 12(2), 0171214, https://doi.org/10.1371/journal.pone.0171214.
Haider, G., D. Steffens, C. Müller, and C. I. Kammann, 2016: Standard extraction methods may underestimate nitrate stocks captured by field‐aged biochar. Journal of Environmental Quality, 45(4), 1196–1204, https://doi.org/10.2134/jeq2015.10.0529.
Heikkinen, J., and Coauthors, 2021: Chemical composition controls the decomposition of organic amendments and influences the microbial community structure in agricultural soils. Carbon Management, 12(4), 359–376, https://doi.org/10.1080/17583004.2021.1947386.
Kammann, C. I., and Coauthors, 2015: Plant growth improvement mediated by nitrate capture in co-composted biochar. Scientific reports, 5(1), 1–13, https://doi.org/10.1038/srep11080.
Schmidt, H. P., C. Kammann, N. Hagemann, J. Leifeld, T. D. Bucheli, M. A. Sánchez Monedero, and M. L. Cayuela, 2021: Biochar in agriculture–A systematic review of 26 global meta‐analyses. GCB Bioenergy, 13(11), 1708–1730, https://doi.org/10.1111/gcbb.12889.
Tammeorg, P., A. Simojoki, P. Mäkelä, F. L. Stoddard, L. Alakukku, and J. Helenius, 2014: Short-term effects of biochar on soil properties and wheat yield formation with meat bone meal and inorganic fertiliser on a boreal loamy sand. Agriculture, Ecosystems & Environment, 191, 108–116, https://doi.org/10.1016/j.agee.2014.01.007.
HOW TO CITE THIS ARTICLE:
Peltokangas K., N. Pitkänen, S. Kanerva, and M. Pihlatie, 2023: Release, retention, and availability – towards mechanistic understanding of nitrogen retention by biochar, FMI’s Clim. Bull. Res. Lett., 5(1), 9–11, https://doi.org/10.35614/ISSN-2341-6408-IK-2023-03-RL
CITATION INFORMATION:
Authors: Kenneth Peltokangas, Nelli Pitkänen, Sanna Kanerva, Mari Pihlatie
Received: Oct 29, 2022
Accepted: Jan 23, 2023
First online: Feb 2, 2023
Published: May 15, 2023
Journal: FMI’s Climate Bulletin Research Letters
Volume: 5
Issue: 1
Pages: 9–11
DOI: https://doi.org/10.35614/ISSN-2341-6408-IK-2023-03-RL
This article is part of the ACCC Special Issue.
Header image: Adobe Stock